PROJECT
DESCRIPTION
RESULTS OF PRIOR NSF SUPPORT
Harold P. Batchelder: GLOBEC: Effects of Seasonal and
Interannual Variability on Zooplankton Populations in the California
Current System Using Coupled Biophysical Models [OCE-0003273 — $140,378
OSU Amt. Only. (April 1, 2000 - March 31, 2005) We
developed coupled physical-ecosystem models of the California
Current system and linked those models with particle tracking
individual-based models (IBMs) of mesozooplankton to examine how circulation
interacting with behavior and physiology impacts the distribution and
population success of major CCS zooplankton species. Biophysical simulations of the ocean off
central Oregon
were run in both 2D and 3D representations. All vital rates were included in the
IBMs. Publications resulting from this work so far include:
Batchelder, H. P., C. A. Edwards, and
T. M. Powell. 2002.
Individual-based models of copepod populations in coastal upwelling
regions: implications of physiologically and environmentally influenced diel
vertical migration on demographic success and nearshore retention. Prog. Oceanogr., 53, 307-333.
Batchelder, H. P., and T. M. Powell.
2002. Physical and Biological Conditions and Processes in the Northeast
Pacific Ocean. Prog.
Oceanogr., 53, 105-114.
Batchelder,
H.P. Forward-in-Time and Backward-in-Time Trajectory (FITT/BITT) modeling of
particles and organisms in the coastal ocean. Submitted to Jour. of Atmos. and Oceanic
Tech.
Perry,
R. I., H. P. Batchelder, D. L. Mackas, S. Chiba, E. Durbin, W. Greve and H. M.
Verheye. 2004. Identifying global synchronies in marine
zooplankton populations: issues and
opportunities. ICES J. Mar. Sci.,
61, 445-456.
James J. Bisagni: (JJB-1): GLOBEC: Satellite-Derived
Estimates of Mixing Across Sea Surface Temperature Fronts in the Georges Bank
Region, (Principal Investigator), NSF/NOAA US GLOBEC Program, 1998-2001,
$149,986. (NSF Award OCE-9806376). This data analysis project assembled a
multi-year (1985-2001) time series of SST frontal locations produced from cloud-cleared
AVHRR satellite images to estimate the mean seasonal cycle.
Bisagni,
J. J. and K. W. Seemann, 1999. Visualizing annual and interannual sea surface
temperature variability over the Gulf of Maine/Georges Bank region. Eos, 80:OS264
Mavor,
T. P. and J. J. Bisagni, 1999. Seasonal variability of satellite-derived sea
surface temperature fronts in the vicinity of Georges Bank.
Eos, 80:OS167
Bisagni,
J. J., K. W. Seemann, and T. P. Mavor. 2001. High-resolution satellite-derived
sea surface temperature variability over the Gulf
of Maine and Georges
Bank region, 1993-1996. Deep-Sea
Research II, 48:71-94.
Mavor, T. P. and J. J. Bisagni, 2001. Seasonal variability of sea
surface temperature fronts on Georges Bank. Deep-Sea Research II, 48:215-244.
JJB-2: Collaborative
Research: GLOBEC-01:Patterns of energy flow and utilization on Georges Bank, (Co-investigator;
D. Gifford, Principal Investigator), NOAA/NSF, 2002-2006, $127,593. (NSF Award
OCE-0217122). The project is in the third of four years. This
project synthesizes key aspects of production and energy flow on Georges Bank, based on US-GLOBEC studies, and augments
the US-GLOBEC data with information from other sources on production processes
at the lower and upper levels of the food web on decadal time scales.
Comparisons of food requirements with inputs from the microbial web indicate
that (1) piscivore needs are relatively constant, even though there are major
shifts in fish species, and these needs can be met by the production of pelagic
juvenile pre-recruit fish. (2) Averaged over the four temporal stanzas, the
needs of the planktivores account for 80% of zooplankton production, but recent
large increases in pelagic fish stocks would appear to leave no food source for
invertebrate predators such as gelatinous zooplankton. (3) Benthivorous fish
requirements are a small fraction (5-15%) of available food, implying that
benthic invertebrate predators such as crabs and shrimp, must play a large role
in the food web. Dynamic models of the fish community include a
predator-mediated shift from benthivorous fish in the 1980s to planktivorous
fish in the 1990s. One postdoctoral scientist at NMFS, Woods Hole and one
graduate student at the University of Massachusetts, Dartmouth
were supported by the award.
Presentations describing the research were given at AGU Ocean Sciences Meeting, Honolulu, Hawaii (February, 2002), the
Inter-American Institute Small-Grant Program Workshop on Bio-physical Modeling
of the Northern Humboldt Current, Valparaiso, Chile (January, 2003), and NOAA
CAFE Workshop, Woods Hole, Massachusetts (June, 2003). Results of the modeling
effort were reviewed in an invited presentation to the opening meeting of the
EUR-OCEANS Network of Excellence Program (Paris, April 2005) and will be
presented at the ICES Annual Science Meeting, Aberdeen (September, 2005). A series of publications is planned for
submission to Progress in Oceanography
within the next 12 months.
Publications to date supported by the research:
Bisagni, J. J., 2003. The seasonal cycles of nitrate supply and
potential new production in the Gulf
of Maine and Georges
Bank regions. Journal of
Geophysical Research, 108(C11), 8015, doi:10.1029/2001JC001136.
Steele,
J.H. and J.S. and Collie 2003. Functional diversity and stability of coastal ecosystems.
In: The Sea, Vol. 13. A.R. Robinson
and K.R. Brink (eds.). In press.
JJB-3: GLOBEC-01:
The physics of Georges Bank and its impact on
biology,
(Co-investigator; R. Beardsley, Principal Investigator), NOAA/NSF, 2002-2005,
$104,863. (NSF Award OCE-0227679). This data analysis project
is focusing on IAV in the magnitudes and extents of the shelf water-slope-water
front and Scotian shelf water cross-overs (SSCs) located on the northeast peak
and southern flank of Georges Bank.
Wishner, K. F., D. J. Gifford, B. K. Sullivan, J. J.
Bisagni, D. M. Outram and D.
D. Van Keuren, 2003.
The biological signature of Scotian Shelf Water crossovers on Georges
Bank during spring 1997. Journal
of Geophysical Research, 108(C11), 8014, doi:10.1029/2001JC001266.
Bisagni, J. J., 2004. Seasonal and interannual variability of the shelf
water-slope water front between 75° and 50° W. Eos, 84:OS80
Bisagni, J. J., H-S. Kim, and K. F. Drinkwater (submitted) Observations and modeling of shelf-slope front seasonal
variability between 75° and 50° W. Deep-Sea
Research II.
JJB-4: Research Experiences for
Undergraduates (REU) Supplement to Award No. ANI-0124945 (Internet2 Connection for the University of Massachusetts Dartmouth’s School
for Marine Science and Technology, (Co-investigator; W.
Brown, Principal Investigator), NSF, 6/1-9/30/2003, $9,548.. This REU Supplement was obtained
in order to provide introductory material to two REU students and allow the
students to connect a Linux server located in Dr. Bisagni's Oceanographic Remote-Sensing
Laboratory (ORSL) with a similar server located in the Remote Sensing
Laboratory of Dr. Andrew Thomas, University
of Maine, Orono, Maine,
using Internet2 technology.
Avijit
Gangopadhyay: Award No. ANI-0124945 (Internet2 Connection for the University of
Massachusetts Dartmouth’s School for Marine Science and Technology,
(Co-investigator;
W. Brown, Principal Investigator), NSF, 6/1/2002-9/30/2003, $150,000. provided
expertise to help set up Internet2 technology at SMAST for large datasets and
model output transfer between modeling groups at SMAST, Harvard, JPL/NASA and
JHU/APL.
Dian J. Gifford: (DJG)-1: Collaborative Research: GLOBEC-01:Patterns of energy flow
and utilization on Georges Bank, Principal
Investigator: D. Gifford, with J. Steele, J. Bisagni, J. Collie, E. Durbin, B.
Sullivan, M. Sieracki, M. Fogarty, D. Mountain, J. Link and D. Palka, Co-Is.
OCE 0217399. 2002-2006, $507,382
(URI component). Results summarized
in Bisagni’s section above.
DJG-2: Collaborative Research: Initiation and Maintenance of Population Maxima
of the Ctenophore Mnemiopsis leidyi in Northern Coastal Waters.
Co-Investigator with B.K. Sullivan, Principal Investigator and J.H. Costello,
Co-Investigator. OCE 0115177 (BKS and DJG)
($655,401), 2001-2004. In situ rate processes relevant to ctenophore population
dynamics and bloom initiation and maintenance were monitored weekly at three
stations for two complete seasonal cycles, together with actual population
response to changes in ambient physical and biological parameters. New and
compelling hypotheses were developed concerning conditions that are critical
for triggering rapid population growth of M.
leidyi. Hydrographic regimes
that determine population distributions of M.
leidyi during the winter months prior to bloom initiation, transport to
regions where ctenophore blooms are initiated in spring, and periods of intense
warming and water column stratification all appear to control the timing and
magnitude of ctenophore blooms. Significant revision of our previous understanding
of the species’ biology includes new insight into physiological limits,
particularly feeding and reproduction at low temperature. Plankton distribution
and abundance data have been added to the URI Plankton Time Series web site
(http://www.gso.uri.edu/phytoplankton/). Three graduate and 6 undergraduate
students were supported by the award. Presentations were made at the ASLO
summer meeting, Victoria, BC (2002), ICES 3RD International
Zooplankton Production Symposium, Dijon, Spain (2003) (3 presentations), and
ASLO winter meeting, Salt Lake City, UT (2003). Publications to date resulting
from the research:
Sullivan, Lindsay J, and D.J. Gifford (2004) Diet of the larval
ctenophore Mnemiopsis leidyi
A.Agassiz (Ctenophora, Lobata). J. Plankton Res. 26: 417-431.
Costello, J.H. and H.W. Mianzan. 2003. Sampling field distributions of Mnemiopsis leidyi (Ctenophora, Lobata):
planktonic or benthic methods? J. Plankton Res. 4: 455-459.
Costello, J.H., B.K. Sullivan, D.J. Gifford, D. VanKeuren and L.J. Sullivan.
Seasonal refugia, shoreward thermal amplification and metapopulation dynamics
of the ctenophore Mnemiopsis leidyi in
Narragansett Bay, RI, USA.
Limnol. and Oceanog. Submitted.
Sullivan, B.K., J.H, Costello and D.J. Gifford. A mechanism linking climate change and
trophic mismatch in coastal plankton. Nature.
Submitted.
1. Introduction, Motivation, and
Background
This proposal addresses several mechanisms by which remote
(basin-scale) forcing is likely impact the population dynamics and production
of Calanus finmarchicus in the coupled Georges Bank/Gulf of Maine (GB/GoM) system.
Variability in the winter North Atlantic Oscillation (NAO) index is related to
changes in various physical and biological parameters across the North Atlantic
and on Georges Bank, but the mechanisms
underlying those relationships are not well known. Understanding basin-to-Bank
connections is important for interpreting patterns of variability observed on
Georges Bank during the core GLOBEC study period (1993-1999) and from earlier
observations (e.g., MARMAP, CPR), and inferring process, whether local or
remote, from those observed patterns. The proposed
research is focused on: (1) zooplankton population dynamics as it relates to
basin-scale climate forcing and physical/biological variability in GB/GoM; (2)
determination of whether basin-scale forcing on the regional ecosystem occurs
through a bottom-up trophic cascade (nutrients->primary production->C.
finmarchicus) or through a more direct supply of seed C. finmarchicus
stock to regional overwintering locations and subsequent transport into GB/GoM;
and (3) evaluation of potential effects on the GB/GoM system of projected
trends and variations in North Atlantic climate.
In order to
address these objectives, we will:
(i)
analyze selected satellite and in-situ
data sets collected during the GLOBEC Georges Bank period;
(ii)
simulate basin-scale circulation fields for the 1990s GLOBEC study
period using an eddy-resolving ROMS already in use and under validation, and
(iii)
conduct a series of coupled biophysical numerical experiments to
test a set of hypotheses on the remote forcing of C. finmarchicus supply and productivity in the NWA/GB system.
We will use newly compiled datasets on nutrient
concentrations and Calanus abundances from the western North
Atlantic to examine potential climate-related mechanisms
influencing Calanus productivity and population dynamics in the GoM/GB.
1.1 Life History of Calanus finmarchicus. The early life history stages and copepodids of mesozooplankton,
especially copepods like Calanus
finmarchicus, Pseudocalanus spp.
and Centropages sp., are major prey for the larvae of
economically valuable, historically harvested groundfish species (cod, haddock)
on Georges Bank. In early spring, C. finmarchicus are by far the dominant
component of mesozooplankton biomass on Georges Bank. However, C. finmarchicus are not year-round residents on the shoals of the
Bank. Each year C. finmarchicus must recolonize Georges Bank from nearby “overwintering”
diapause (usually as C5) populations in deep regions (GOM basins,
Scotian Shelf basins; slope waters SE of Georges Bank). In the Gulf of Maine,
C.
finmarchicus’ return to the surface begins around December,
with the last individuals exiting diapause a few months later. Awakening C5s arrive to the
surface and mature to the adult stage.
They use the plentiful resources of the spring phytoplankton bloom and
the microzooplankton that accompany it to produce many clutches of eggs that
are broadcast into an environment favorable for individual feeding and
growth. Due to the abundant
phytoplankton on the Bank in late winter and spring, many grow and develop
rapidly and survive to the late copepodite stage. At the C5 stage, some mature
to adults and produce a next generation within the same year; but others,
instead of maturing and reproducing delay maturation and accumulate stores of
energy-rich lipids. Eventually, as
conditions warm, the C. finmarchicus
(usually as C5) depart the warming surface waters in early summer
(May-June) to deeper waters. If
this vertical descent occurs in shallow regions (<300m) like Georges Bank, it is likely that those individuals
die—probably to demersal predation.
To complete their life cycle, these diapausing individuals, who are only
marginally active with low metabolic rates (Ingvarsdottir et al. 1999), remain at depth until they are cued to exit this
quiescent state and return to the surface to feed during the next year’s
spring bloom. This diapausing state
is often misnamed as an “overwintering” phase, when in fact, it is
a strategy to survive the “summer,” and should more appropriately
be termed an “oversummering stage.” Calanus finmarchicus has a life history strategy that enables
individuals in the population to exploit (for reproduction and somatic growth)
the large spring blooms that occur in temperate and boreal ocean systems, yet
avoid the warm, low-food conditions of summer and autumn that would result in
rapid consumption of their stored lipids.
Interannual and interdecadal variations in the overall productivity of
the coupled GB/GoM ecosystem and adjacent slope sea waters, as reflected by
variations in the abundances or biomasses of C. finmarchicus, or altered seasonality in the production cycles
that support their production (Greene et
al. 2004), may strongly influence survival and eventual recruitment of
planktonic larval cod and haddock. Knowledge of copepod prey field responses to
water mass and lower trophic level variability is important to understand why
depleted cod and haddock populations on Georges Bank are recovering or not.
1.2 A Spawning Stock Size Approach to Calanus finmarchicus. For a
century, fisheries biologists have documented strong impacts of fish stock
sizes on subsequent recruitment (the so-called stock-recruit relationship). We believe such an approach is equally
valuable in considering interannual variability in C. finmarchicus
population dynamics and abundances.
A general view of the Georges Bank
program has been that the population dynamics of C. finmarchicus are predominantly controlled by what happens within
the 100m isobath of the bank (US GLOBEC 1992; p. 9). In later years of the project it was
recognized that the adjacent deep water regions in the Gulf of Maine
are important, but they were only sampled during the core January-June period
(some limited sampling was done in October and December of 1997-99). We argue here that the “spawning
stock” of C. finmarchicus,
e.g., the abundance of deep-dwelling diapause individuals in the basins of the
Gulf of Maine and Scotian Shelf, and in the slope water region SW of Georges
Bank in summer and autumn, at the beginning of the short productive
winter-spring is equally as important as the population dynamics that occur
within the confines of the 100m isobath on Georges Bank. Our population models coupled with
transport models will be used to address the potential for interannual and
interdecadal variation in C. finmarchicus
“spawning stock” to influence subsequent dynamics and abundances on
Georges Bank.
1.3 Impact of Climate-related Variability on Calanus finmarchicus. In
the Northeast Atlantic there is substantial evidence that Calanus finmarchicus population abundances have varied inversely
with the North Atlantic Oscillation (Planque and Reid, 1998; Greene et al.
2003), but C. finmarchicus abundance in the Gulf of
Maine during 1960-90 covaried directly with the NAO (Conversi et al. 2001), suggesting that perhaps
different mechanisms, acting through temperature and ocean circulation
patterns/intensities are operating on the eastern and western sides of the
North Atlantic. However, Planque
and Reid (1998) also document the sometimes ephemeral nature of such
climate-ecosystem relationships, as they observed the pattern for the Northeast Atlantic breakdown in recent times. Some of the approaches and issues
related to identifying patterns of zooplankton response to climate variability
are discussed in Perry et al. (2004).
In their investigation of long-term temperature and
salinity variability on the
Scotian Shelf and in the Gulf
of Maine, Petrie and
Drinkwater (1993) demonstrated that the dominant low-frequency event over the
last 45 years was the cooling and subsurface freshening of water masses between
1952 and 1967, followed by a rapid reversal of these trends. Largest declines
in temperature (4.6oC) and salinity (0.7) occurred near 100-m depth
over the continental slope and were attributed to increased westward transport
of the Labrador Current past the tail of the Grand Banks and into the Slope Sea
along the shelf break. The GLOBEC decade (1990s) of studies in the
Northwest Atlantic (Georges Bank) ecosystem
was generally a period of high NAO index (Fig. 1). The major exception to this pattern
occurred in 1995-96, when the NAO index flipped from being strongly positive to
one of its most negative values of the 20th century (Greene and
Pershing, 2000). This reversal was
short-lived, however, as by 1998 the NAO was positive again and remained so
throughout the decade.
There
was no apparent immediate and dramatic response of the plankton community in
the GoM/GB region to the 1995-96 NAO reversal. Bisagni et al. (2001) document significant cooling of adjacent slope water
surface temperatures (using AVHRR) from 1994-1996, which covaried with volume
transport anomalies through the Northeast Channel. Calanus finmarchicus populations on Georges Bank had slightly
slower development and later maturation times due to lower temperatures in 1996
than in 1995 (Durbin et al.
2000).
More
significant impacts on the plankton community were observed in the summer of
1998. During
the 1970s, ‘80s, and early ‘90s, conditions over the Scotian Slope
and in the deep basins of the Scotian Shelf and Gulf of Maine
remained relatively warm and saline compared to the previous two decades.
However, during early 1997, moored instruments at the shelf break off St.
Pierre Bank, south of Newfoundland,
detected the arrival of a sustained pulse of cold, fresh Labrador Slope Water
(LSW) at depths of 50-400 m. During
the rest of that year and early into 1998, the LSW progressed along the shelf
edge to the mouth of Northeast Channel (Jan.’98) and into the
Mid-Atlantic Bight (Mar.-Apr. ’98; Drinkwater, et al. submitted).
Passing through channels and gaps in the outer banks, the cold, fresh
waters flooded the inner basins, changing the temperatures and salinities
abruptly by the order of 4.0oC and 1.0 psu, respectively. By August 1998, the LSW had filled all
the inner basins of the Gulf
of Maine below depths of
100 m. This influx of cold,
nutrient-poor northern water into the Northwest Atlantic (NWA), even into the
GOM itself, was accompanied by a significant decline in the abundance of
diapausing Calanus finmarchicus in
the GOM in fall 1998 (Fig. 1; Greene et
al. 2003). This decline might
have been an effect of the generally lower productivity due to the lower
nutrient concentrations of LSW, or it might have been due to altered
circulation patterns associated with the presence of the LSW.
1.4 Mechanisms for Climate-related Variability of Calanus Spawning Stock
Size in NWA. Interdecadal variability (e.g., 1960s cold
anomaly; warm 1970s) in Western Boundary Current (WBC) transports (Petrie and
Drinkwater 1993; Drinkwater et al.
unpubl.) can create large changes in ocean temperature, particularly in deeper
waters, that alter the distribution, migrations, spawning times, growth and
physiological rates of resident organisms.
The massive kill of tilefish along the Mid-Atlantic Bight in the early
1880s may be an extreme example of the impacts from an excessive inflow of cold
subpolar water (Marsh et al. 1999).
Altered temperature is likely to impact the physiology of diapausing C. finmarchicus and disrupt the timing
of diapause exit, for which the cues are unknown. If so, this could impact the synchrony
of C. finmarchicus return to the
surface relative to the seasonal production cycle and phytoplankton blooming.
Increased equatorward advection along the shelf-slope should provide enhanced
southward transport of organisms, and this may lead to export of Calanus finmarchicus from the Labrador
Sea to the Slope Sea, directly as diapausing stages in
deep inflows, or indirectly through southward Labrador Shelf surface transports
(Head et al. 2003). C.
finmarchicus from the Labrador Sea may be important in seeding the Scotian
Shelf, which is upstream from the Gulf
of Maine and Georges
Bank. Diapause depths of C.
finmarchicus vary across the North Atlantic, with shallowest depths
(<1000m) in the western Atlantic. In Wilkinson Basin in the Gulf of Maine
diapausing Calanus are between 170
and 250 m, or up to 70 m off the bottom, and abundances in December 1999 were
about 20,000-30,000 m-2; there is evidence that 1998 diapausing
populations were less abundant (Greene et
al. 2003; M. Benfield, pers comm).
Discrete depth sampling from slope waters off southern New
England show diapausing C.
finmarchicus at 500 m, with 50% of the population between 400 and 600 m
(Miller et al. 1991). C.
finmarchicus C5 densities in diapause ranged from 2000-12,000 m-2,
with a peak in October-November. C. finmarchicus diapause at depths of
300-900 m off Atlantic Canada and in the southwestern Labrador Sea (Head, pers
comm.); diapausing copepodite densities off Labrador in December 2002 were ca.
10000-20000 m-2. During
spring and early summer C. finmarchicus
comprised >60% of the biomass of the mesozooplankton and >80% of the
abundance of large copepods of the Labrador Sea
(Head et al. 2003). Average surface (0-100m) C. finmarchicus abundance in the western
and central regions of the Labrador Sea was
17000 m-2 during the same time. Strong southward flows from the Labrador Sea could export large numbers of Calanus to the
NW Atlantic. As an extreme example,
during the 1998 event the arctic-boreal copepod C. hyperboreus was recorded south of Georges Bank
for the first time in more than 30 years of CPR sampling (Johns et al. 2001). A third effect of the change in WBC
transport is on nutrient concentrations (Petrie and Yeats 2000). Labrador Slope Water has about one-half
the nutrient concentration of older Warm Slope Water (WSW), which is more
commonly found adjacent to the slope in the western North
Atlantic. The low
nutrient content of LSW will directly affect biological productivity in the Gulf of Maine
and Georges Bank since deep water influx through
the NE Channel is the major source of nutrients supporting the early spring
productivity of the GoM/GB system.
1.5
Multi-Decadal to Interannual Variability of Basin-scale Circulation Related to
NAO. Recent studies (Hoerling et al. 2001; Hurell et al., 2001) show that the NAO has a major impact on the North Atlantic circulation and variability. Taylor and
Stephens (1998) show that over the period 1966-96, the position of the Gulf
Stream north wall (GSNW) appears to be determined by the NAO, but with a lag of
about 2 years. They attribute the lag to propagating Rossby waves (Gangopadhyay
et al. 1992), and suggest that the
delayed-response predictability observed for the 1977 to 1988 period has been a
feature of the Gulf Stream system for last 30
years. Figure 2 shows the Gulf Stream position
(position of the 15oC isotherm from data in Parker et al., 1995) overlaid with winter NAO
from Drinkwater (pers. comm.). Both Gulf Stream
position and NAO index exhibit an upward trend since 1970 (Conversi et al. 2001; Taylor and Gangopadhyay
2001). There was a downward trend from 1950 to 1970, suggesting that these
trends may be due to climate variability. The Gulf Stream
was furthest south during the 1960s, and furthest north during the 1950s and
1980s. The Gulf Stream position exhibits
pronounced interannual variation at periods of 3-5 years.
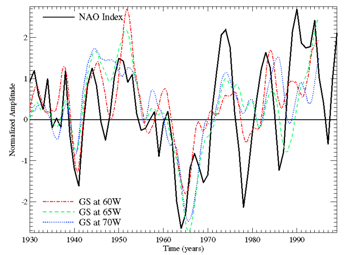
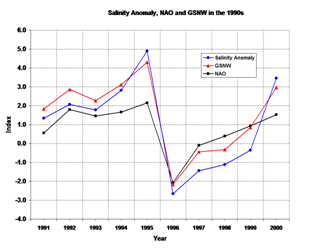

Fluctuations of the Gulf
Stream are correlated with the NAO index, although there seems to
have been a phase shift since the 1960s. Temperature and salinity variability
in the GoM/GB region during the 1990s has been well documented. Mountain (2004)
analyzed the temperature and salinity in NAFO subareas 5 and 6 during the 1990s
and discovered that the surface waters in the GoM/GB region underwent a gradual
freshening (Figure 3) when compared to an earlier period (1978-87), due to an increase inflow of Scotian
Shelf Water. Drinkwater (2004) investigated atmospheric and sea-ice conditions
in thenorthwest Atlantic during 1991-2000 and
related them to low and high-NAO conditions. The atmospheric low-pressure
system over the northwest Atlantic shifted eastward during the latter half of
the decade, causing weaker northwesterly winds, warmer temperatures in the
Labrador Sea to the Gulf of Maine and a reduction of sea-ice.
Fratantoni and Pickart (2005, submitted ms.) analyzed twelve years
(1990-2001) of hydrographic data to examine alongstream evolution of the
shelfbreak front in the western north Atlantic from the west coast of Greenland
to the Mid-Atlantic Bight.. They found that the shelfbreak front in the
Labrador Sea is composed of two fronts: one inshore front with fresher Labrador
Sea water, and the other offshore with Irminger Sea
water. This latter front completely disappears near the Grand
Banks. They also found that during the 1990s there was an
alongstream freshening of the shelfbreak flow right after the tail of the Grand
Banks, even before the front is joined by the freshwater plume outflow from the
Gulf of St. Lawrence. They suggest that the
outer “salty” portion of the front turns offshore at the tail of
the Grand Banks and the fresher surface layer
follows the shelfbreak towards the GoM/GB region.
These three studies raise questions regarding the source of the
low-salinity waters in the Gulf
of Maine in the 1990s. Is
it the fresh surface layer of Labrador Sea Water that flows along the shelf
break and enters the GoM/GB region? What contribution, if any, comes from the
freshwater outflow from the Gulf of St. Lawrence?
What is the large-scale response of the shelfbreak system to seasonal forcing?
What is the basin-scale response of the shelfbreak system to interannual
NAO-like forcing? Our numerical modeling will focus on these questions by
analyzing the basin-scale four-dimensional fields. Figure 3 shows the
decade-long time-series of the NAO, the salinity anomaly in the GoM/GB region,
and the GSNW Index from Taylor and Stephens (1998). It is evident that when the
Gulf Stream was further south, the Gulf was
fresher, and vice versa. The correlation between the salinity anomaly and the
GSNW is 0.78; while that between the salinity anomaly and the NAO is 0.64. Our
model simulations will provide dynamical insights on these correlations.
1.6 Ongoing Basin-scale Modeling for Simulating High and Low NAO Forced
Fields.
The physical modeling
component is based on ROMS (Regional Ocean Modeling System), which was
developed and modified by Rutgers
University, UCLA and
NASA/JPL scientists. ROMS is a
split-explicit, free-surface, terrain-following ocean model, where short time
steps are used to advance the surface elevation and barotropic momentum, and
larger time steps for temperature, salinity, and baroclinic momentum. Recent
modifications include subgrid-scale
parameterizations (Gent and McWilliams 1990; Danabasoglu et al. 1994; Griffies et al.
1998) and sigma-coordinate
pressure-gradient error reduction
(Shchepetkin and McWilliams 2003). The improved time-stepping
algorithm (Shchepetkin and McWilliams 2004)
provides improved run-times.
Using NASA Interdisciplinary Science
(IDS) funding (NASA NNG04GH50G), we purchased an Altix 350, eight-processor SGI
computer for ROMS modeling. The eddy-resolving 15-20km ROMS has been configured
for the North Atlantic (Figure 4). It
encompasses the Gulf Stream system, the coastal Gulf
of Maine, Georges Bank, Gulf of St.
Lawrence, Labrador and Irminger seas, and the
shallow regions near coast where the depth is less than 50 meters. The
resolution varies from 10 to 15km (north) to about 20-22km in the southern
tropical region and there are 50 vertical levels.
For NASA, we are carrying out two
10-year-long simulations representative of the two phases of the NAO. We have
started the simulation for the high-NAO period. The initial
temperature-salinity fields are from Levitus and the forcing fields are based
on the annual climatology, computed for 1980-93, by the Southampton
Oceanographic Center (SOC). The model is (as of 7 May 2005) on its sixth year of simulation and
the results are being analyzed.
In summary,
studies have shown correlations among the basin-scale NAO index and the
circulation variability realized by Gulf Stream position, Slope
Sea and Labrador Slope water
properties, as well as between the NAO and C.
finmarchicus populations in the western North Atlantic.
However, additional kinematical and dynamical studies are required in order to
understand the processes underlying these relationships. We are simulating
ocean conditions in the North Atlantic for
high- and low-NAO periods under a different project. Thus, in this proposal,
our focus will be on 1) simulating the GLOBEC field years (1993-1999), and 2)
using the results from all three periods to force a Lagrangian model of Calanus finmarchicus. We will conduct a
number of “synthesis experiments” focused upon C. finmarchicus.
2. Research Objectives,
Questions & Hypotheses
2.1 Climate-Related Basin-Scale Circulation
Variability Questions, Two distinct time-scales are important
for evaluating the impact of climate and basin-scale forcing on the GoM/GB
ecosystem. The first is the multi-decadal variability (Petrie and Drinkwater
1993) which results from the accumulated effects of a sustained high-NAO or
low-NAO condition. Clearly, the C. finmarchicus population responds to
sustained long-term changes in the system. Our present NASA modeling effort will address this with
the addition of biological modeling.
Rossby (1999) attributes the annual north-south
shifting of the eastward-flowing Gulf Stream
to a time-varying input of water from the Labrador Shelf. An increasing volume
transport of Labrador shelf water during 1996-97 (Smith etal. 2001) might push the Gulf Stream
further south. Associated with this
shift is westward penetration of LSW along the shelf break (Fig. 5), which is
also evident as cooler SST (Fig. 6). Separation of the Gulf
Stream from the coast may be set-up by the wind stress curl in the
subtropical gyre, while the latitudinal excursion of the eastward flowing GS
(east of 65W) is related to the amount of water flowing in from the Labrador
Shelf and/or slope, which in turn might be linked to NAO variability.
We will peform data analysis, modeling, and diagnostic
analysis, to address several scientific questions about the NAO and its impact
on western North Atlantic circulation and its
variability:
(1) What is the
interannual variability of the kinematical relationship between features such
as the north wall of the Gulf Stream, Gulf Stream
warm-core and cold-core rings and shelf/slope front, Labrador Slope Water, and
Labador Sea Water?

(2) What is the
nature of the variability of these features during the 1993-1999 US-GLOBEC
measurement period? It is presently unclear how the salinity anomaly occurred
during the 1990s. The high correlation between the freshening of the GoM/GB
region with southward movement of the Gulf Stream
(Figure 3) is compelling enough to look for large-scale thermohaline forcings
following Rossby and Benway (2000). The shelf water influx (Mountain, 2004)
points to increased ice-melt.
(3) What is the nature of the
interactions between the subtropical gyre and the subpolar gyre in simulations
conducted for high-NAO years versus low-NAO years? Their interaction through
the deeper waters in the thermohaline circulation may be very important for
determining the fate of Calanus finmarchicus being
transported from Labrador Sea to the Slope sea
region.
2.2 Climate-Related C.
finmarchicus Variability Questions. A goal of the proposed
research is to examine the impacts of seasonal and interannual variation in
ocean conditions in the western North Atlantic (Labrador Sea, Slope Sea
and GB/GoM) on productivity and seeding (supply) of Calanus finmarchicus to the GB/GoM. We will develop Lagrangian individual
and behavior based models (IBMs) of C.
finmarchicus growth, development and transport. Using these models we will evaluate potential
physical exchange of copepods between the semi-enclosed Labrador Sea and Slope Sea
gyres, and between the Slope
Sea and the GB/GoM. There
is an interesting conundrum associated with the MERCINA (2001) hypothesis on
how the Labrador Slope Water influences the Slope Sea
and supply of Calanus to adjacent
continental shelves. The puzzle is
that when Labrador Sea water masses are significant off the Scotian Shelf,
Georges Bank and in the Slope Sea region, C.
finmarchicus populations in the Gulf of Maine tend to be low
(Figure 1). This is counter to expectations; given
the high C. finmarchicus abundances
in both surface layers (during spring-summer) and deep diapausing layers
(remainder of year) in the Labrador Sea, one might expect higher C. finmarchicus seed supply when
Labrador influences are great in the Slope Sea region. The discrepancy may be
related to temperature of the water that the diapausing stocks are in, and the
role that temperature-controlled physiological processes have on terminating the
diapause stage. Head et al. (2000)
report that surface waters of the Southern Labrador Sea
in May and June have a large fraction of adult females that are from awakened
diapausing C5s. This
suggests that in the Labrador Sea, the alarm clock for waking up diapausing C. finmarchicus is much later (by up to
5 months) than the waking time in the Gulf of Maine and Slope Sea (usually
December-February; Durbin et al.
2000). If the arousal time is
intrinsic to the individual, and if this behavioral habit (pattern) is
transported with the diapausing individuals at depth, then imported Labrador
Sea copepods will awake too late to exploit the spring bloom in the NW
Atlantic. We will test the hypothesis that
basin-scale climate oscillations (NAO), operating through time-varying
intensity of deep and intermediate water-mass formation and ocean circulation,
result in shelf- and slope-water transports and fluxes of Calanus and nutrients that are advantageous at some times and
disadvantageous at others for the development of large overwintering
populations of C. finmarchicus in
deep waters adjacent to the GB/GoMsystem.
The
core hypothesis and predictions of this work are:
Hypothesis: The
occurrence of large populations of Calanus
finmarchicus in the coupled GB/GoM system REQUIRES (1) high seed stocks
(supply) of diapausing C.finmarchicus
in the deeper ocean regions nearby (GOM basins and the Slope Sea), (2) that the
deep C. finmarchicus stocks terminate
diapause at the appropriate time to be synchronous with continental shelf
spring blooms, and (3) a nutrient enriched, highly productive ecosystem in the GB/GoM
to sustain high growth and survival rates of Calanus that will provide seed for the subsequent year.
Prediction
A: Overwintering Calanus
finmarchicus seed stocks are LOW and GB/GoM productivity is HIGH when the
water masses of the Slope Sea have little influence (input) from
Labrador-Irminger Gyre (Labrador Slope Water) water masses (due to the
relatively nutrient replete bottom waters and low Calanus supply in Warm Slope Waters), but C. finmarchicus recruitment is good because of a near-perfect match
between the time of diapause awakening and the time of the spring bloom, the
latter of which is large because of the higher concentration of nutrients in
deep warm slope waters.
Prediction
B: Overwintering C. finmarchicus seed stocks are HIGH
and GB/GoM productivity is LOW when the water masses of the Slope Sea have a
large proportion of Labrador Sea water (due to the relatively nutrient-depleted
bottom waters and high C. finmarchicus
supply in cold Labrador Slope Water), but
recruitment and productivity are poor because of the generally low
springtime productivity (low nutrients) and a timing mismatch between diapause
awakening, ascent and reproduction and the NW Atlantic spring bloom.
3. Research Approach
It is clear that the relationship between the GB/GoM ecosystems
and basin-scale variability related to NAO occurs over at least two different
time-scales: (i) multi-decadal, and (ii) interannual. We propose to carry out a
set of eddy-resolving basin-scale model simulations, analyze satellite and
other available data sets from the GLOBEC period, validate the model
simulations, and use the simulations to address a set of questions that relate
ecosystem variability in the Scotian Shelf, Gulf
of Maine and Georges
Bank regions to fluctuations of the NAO.
Our approach is based on (i) analyzing satellite and
hydrographic observations during 1993-1999 period; (ii) simulating the
basin-scale impacts on Calanus finmarchicus
from an eddy-resolving North Atlantic model simulation of high vs. low NAO
periods; (iii) simulating basin-scale physical and biological fields for the
GLOBEC period (1993-1999); and (iv) synthesizing the model simulations and
GLOBEC data sets during the 1993-1999 period to address the specific questions
outlined in Section 2 above. In addition, we will provide the basin-scale
simulation fields for forcing other regional models (both physical and
biophysical) to specifically address detailed hypothesis related to the
interannual variability of the GB/GoM ecosystems.
3.1 Analysis and Use of
Satellite-derived SST (1993-1999). We propose a
comprehensive synthesis using both daily satellite-derived SST data from the
western North Atlantic collected between 1993
and 1999 and five-day averaged, optimally-interpolated (OI) SST fields also
derived from satellite data. The cloud-free OI SST fields have been
already constructed for the five year period (1995-1999) encompassing the
entire US-GLOBEC Northwest Atlantic Georges Bank field program. Five-day
averaged OI SST fields were produced using the OI method of Chelton and Schlax
(1991) from a time series of approximately 4000, twice-daily, high-resolution
(~1.4-km) cloud-cleared Advanced Very High Resolution Radiometer (AVHRR)
satellite images (Bisagni et al.,
2001). Calibration of the resulting five-day averaged OI SST fields with
five-day averaged bulk temperatures (z » 1-m
depth) from four NOAA data buoys located within the domain was completed using
a least-squares adjustment between mean satellite-derived SST values from a 3 ´ 3
pixel array centered on each buoy location and buoy temperature values. The
final set of calibrated OI SST fields will be used for our planned
high-resolution synthesis (see Section 3.2.2) within the Gulf
of Maine and Georges
Bank region.
3.2 Basin-Scale Circulation
Modeling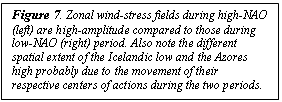
. Two of us (AG and JB) are funded by NASA to simulate
decade long periods of high and low NAO conditions. The high NAO conditions are currently
being simulated. During summer
2005, simulation for the Low-NAO period will be done. Levitus will be used for
initial temperature and salinity fields. Wind forcing is derived from the
ICOADS data set during the sixties (1962-1971, low-NAO period). Zonal
wind-stress fields for winter (January) and summer (July) for high and low-NAO
periods are shown in Figure 7. We
are addressing a hypothesis related to ‘depletion vs. dilution’ of
nutrients, i.e., the dominance of low nutrient Labrador Sea Water influx in the
Slope Sea during low-NAO periods as opposed to high nutrient warm slope water
coming through the Gulf Stream system during high-NAO periods. So, we focus the results of the
simulations towards realistically generating the Gulf Stream System and the
Labrador Sea Water influx into the Slope
Sea. Figure 8 show that
the model is successfully reproducing the Florida Current at 32N, with core
speed of 50cm/sec and a reasonable vertical extent of 800 meters. The transport
of the current with 100km width at the surface is about 40Sv. Figure 4 shows
the cold Labrador water flowing southward in a blue ribbon around the tail of
Grand Banks (Fig. 8 – right panel). We will carry-out targeted
multi-year simulations for the North Atlantic
starting from the results of the 10-year long simulations.
3.2.1 Proposed basin-scale simulations. In the first year, we will integrate the North Atlantic ROMS OGCM
from its final high-NAO state with monthly NCEP reanalysis fluxes during
1988-1999. Based on our experience (Chao et al., 1996), we start the simulation
five years before the start of GLOBEC (1993) is to allow the winds to force the
response of the Gulf Stream and Labrador
Current in a time-integrated manner. The simulated fields for 1990 through 1999
will be used for the zooplankton model to generate C. finmarchicus distributions in the NWA. Both physical and
biological fields will be analyzed for interannual variability studies and used
in the C. finmarchicus experiments in
the second year.
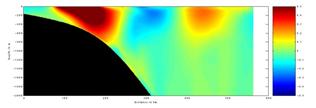
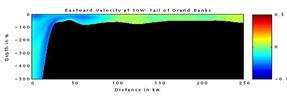
The success
criteria for the proposed physical and biochemical simulation will be our
ability to: (i) reproduce the high-NAO vs. low-NAO behavior of the GS path
similar to that shown in Fig. 4, (ii) reproduce the nutrient variability in the
Slope Sea,
and (iii) simulate specific such as the 1997-98 Labrador Slope Water intrusion
in Slope Sea. Biophysical simulations (described later) will be used to
understand the basin-scale impact of the NAO-driven Labrador
Sea water advection on the slope sea region. In particular, the
vertical distribution and variation of flow and their influence on nutrients
and Calanus in the slope sea will be
compared between 1995 and 1998.
We will investigate transport pathways from the Labrador Sea/Slope
to the Slope Sea
(between the North wall of the Gulf Stream and the shelf break), the transport
from the Slope Sea
to the Labrador Sea and retention within the Slope Sea.
We will explore whether eddies make an important contribution to the retention
in the Slope Sea, transport from the Labrador Sea/Slope to the Slope Sea, and from
the Slope Sea to the Labrador Sea.
We are also interested in whether there are significant changes in the
transport pathways from year to year.
For example, were there changes in the pathways related to the 1996-98
NAO event? How different are these pathways for a high NAO (1994-95) period
from a low-NAO (1997-98) period?
3.2.2 High-resolution field generation using
basin-scale output and GLOBEC data sets. We propose to generate a series of
high-resolution (5-km) fields by melding the basin-scale fields (10-15 km
resolution) with GLOBEC period data sets using multi-scale objective analysis
(OA) as done previously ((Gangopadhyay et
al. 2002, 2003). The OA is performed in two stages (Lermusiaux, 1999a). In
the first stage, the largest dynamical scales (from the basin-scale model
fields) are resolved at each level, using estimated large-scale e-folding
spatial decays, zero crossings, and temporal decay. In the second stage, the
synoptic dynamics of interest (meso-scale and sub-basin scale, from data or
synthetic profiles as explained below) are resolved using its estimated
space-time decays. The background for this second stage OA is the first-stage
OA. The primary assumption made in this two-scale OA is that the errors in the
basin-scale (first-stage) and synoptic (second-stage) dynamical scales are
statistically independent. This procedure effectively and smoothly melds the
synoptic profiles or observational profiles and climatology for the GoM/GB
region. For detail mathematical description of the OA parameters, see
Lermusiaux (1999a,b).
The high-resolution synthesis will be carried out in four
steps. First, the temperature and salinity fields from the basin-scale ROMS
simulation will be averaged over 5-day periods during the GLOBEC period of
1993-1999. The locations for these profiles are shown in Figure 9 as black dots
within
the region of interest (73-50W, 38-46N). Second, the 5-day averaged SST fields
and the ROMS temperature field at the bottom level will be synthesized to
develop synthetic temperature profiles at pre-determined locations (red dots in
Figure 9). The synthesis equation is given by: T(z) = Ts - (Ts
– Tb) Ф(z). Here Ts is the surface
temperature (SST) and Tb is the bottom temperature from
the nearest ROMS
grid point. Ф(z) is the non-dimensional structure function (unity at surface to
zero at bottom) for the temperature profile of the mesoscale feature at the
location of interest. The forms of Ф(z) for all the 270 profiles are
available from Gangopadhyay et al.
(2003). Third, the corresponding salinity profiles will be determined using
appropriate GLOBEC data-derived T-S relationships in conjunction with the T(Z)
derived in step 2. Finally, the mesoscale temperature and salinity profiles
from above two steps will be melded with the basin-scale fields for each five
day period using multiscale OA. This process will generate three-dimensional
high-resolution fields for temperature, salinity and geostrophic velocity every
five-days during the whole GLOBEC period (1993-1999) that has the climatic and
basin-scale impact of NAO in the flow field. We will thus resolve the
sub-mesoscale variability of the Scotian Shelf and the flow through the
Northeast channel into the Gulf of Maine and Georges Bank
system with climate-to-basin scale variability incorporated in the mass and
momentum field. A twin experiment will be carried out to understand the
ecosystem behavior for Winter-Spring-Summer (WSS) of 1995 compared to that
during WSS of 1998 using the IBM modeling as described in section 3.3.
3.2.3 Multi-decadal and Interannual Validation of Simulations. The model simulations will be
validated with data sets available for the Gulf Stream
paths from Gangopadhyay et al. (1992)
and the GSNW indices from Taylor and Gangopadhyay (2001) for last 50 years. The
simulated seasonal water-mass climatology for the Gulf of Maine
region will be validated against MARMAP and GLOBEC data sets (Mountain,
2004). Simulated water-mass climatology for the Labrador
Current and Scotian Shelf region will be validated against archived data sets
(Loder et al., 2001; Fratantoni and
Pickart, 2005) and variations in salinity anomalies in the Labrador Sea during
1950-1998 (Houghton and Visbeck, 2001). Model simulations in the GoM/GB region
will be validated with 1985-2000 SST data from Bisagni’s lab.
3.3 The C. finmarchicus Modeling
Experiments. The biological modeling follows naturally from the analysis of the
physical fields and will be built incrementally from that foundation. The population dynamics of Calanus finmarchicus and transports by
oceanic flows have been extensively modeled in both the Northwest Atlantic
(Miller et al. 1998) and other
regions of the North Atlantic (Tittensor et al. 2003; Bryant et al. 1998; Carlotti and Wolf, 1998; Carlotti and Radach 1996;
Harms et al. 2000). However, almost all of those prior
efforts have emphasized the population dynamics of the C. finmarchicus when they are actively feeding and reproducing in
the near-surface layers. An
emphasis of the coupled biophysical Lagrangian modeling we propose is to
examine the role of transports on supply of deep diapausing populations of C. finmarchicus to the Slope Sea region
from the Labrador Sea, where diapausing populations are very large (>17000 m-2)
at depths of 300-800m (Heath et al.
2004; Head, pers. comm.). The
Labrador Sea is recognized as one of the epicenters of C. finmarchicus in the North Atlantic (Tittensor et al. 2003; Heath et al. 2004), so export of water from the Labrador
Sea to regions further south would be expected to provide influx
of Calanus. Transports of C. finmarchicus from diapausing populations to the North Sea have
been the focus of both field studies and extensively modeled (Heath 1999; Heath
et al. 1999; Heath and Jonasdottir
1999; Gallego et al. 1999), but our
proposed modeling is the first to examine transport of diapausing populations
in the western North Atlantic.
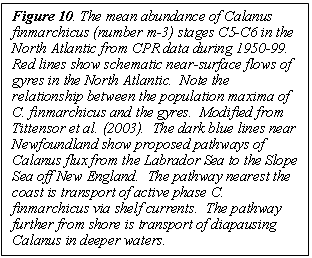
There are three
distinct biological (Calanus finmarchicus)
model experiments, which are linked directly to the physical model
experiments. The first experiment
(done in year 1) will use the results of the decade long simulations of the
“prototypical” high NAO (1980-93) and low NAO (1962-71)
periods. Basin-scale physical
simulations for both high and low NAO periods are currently being done (with
NASA funding), so the velocity and tracer fields will be available in year one
for Lagrangian modeling of Calanus
transports and physiology. The
second biological modeling experiment (year 2), will use velocity and tracer
fields from simulations of the GLOBEC field years (1993-1999) to explore the
impact of the 1996 NAO reversal and later inflow of Labrador Slope Water into
the NW Atlantic on diapausing Calanus
finmarchicus populations.
Comparison of the results of multiyear model simulations from experiment
1 and experiment 2 will provide insights on the effects of a prolonged influence of cold, nutrient
deplete Labrador water on the Slope
Sea, GOM-GB, and Calanus finmarchicus populations. The experiment 1 and 2 simulations on
deep diapausing populations will be complemented by simulations of active phase
Calanus that might be transported
within near-surface continental shelf currents. For these simulations, initial and
boundary Calanus densities for the
Slope and Labrador Sea will be derived from
the Bedford Institute of Oceanography’s Calanus finmarchicus data base (Mary Kennedy, pers comm.). The third biological modeling experiment
(year 2) will use higher resolution velocity and tracer fields derived from
basin-scale physical simulations melded with objectively analyzed SST fields as
described in section 3.2.2. Higher
resolution physical fields for the Scotian Shelf, GOM and GB, will provide the
physical template to examine potential fluxes of Calanus from Slope Waters SE of the continental shelf, through the
NE Channel into the Gulf
of Maine deep basins.
For
each circulation scenario, we will begin with an analysis of the transport
using tracer experiments with Lagrangian models. Note that the high vertical
resolution (50 levels) of the present ROMS set up will provide more accurate
vertical fluxes than many studies before. Biological realism will be added
sequentially. Deep diapausing C.
finmarchicus populations in the southern Labrador Sea will be initiated
with a range of lipid stores; timing of awakening will be related to lipid
consumption at depth (recognizing that most lipid is not consumed during the
diapause phase, but rather remains available for egg production immediately
following maturation) (Irigoien, 2004), and therefore a function of
temperature. Heath et al. (2004) inferred from the assumed
neutral buoyancy depth of diapausing C5 C. finmarchicus in the Labrador Sea that C.
finmarchicus implied lipid weight was ca. 27.5% of total body weight. For models, we will explore a range of
lipid weights that span 20-70%, which encompasses the range of measured lipid
fractions in diapausing C. finmarchicus
(Heath et al. 2004). Initial depths of C. finmarchicus in the Labrador Sea
will vary from 300-1000m (median near 500m; Heath et al. (2004); Head, pers comm.). These simulations will indicate
sensitivity to deviations in diapause depth, temperature, and timing. Simulations
of the active phase (December-May) will require both temperature and food
(chlorophyll) fields as controlling factors for C. finmarchicus development and growth. Satellite-derived chlorophyll fields
from SeaWiFS and CZCS will be used to derive a climatological seasonal
chlorophyll distribution. In the
GLOBEC study region on Georges Bank, satellite
fields will be complemented by in situ observations of chlorophyll. Vertical
distributions of chlorophyll will be generated from local seasonal observations
of vertical chlorophyll distribution, scaled by the value observed by satellite
at the sea surface. The impact of chlorophyll variability will be explored
using SeaWiFS chlorophyll fields from particular years. The biological
simulations will focus on influence of basin-scale circulation variability on C. finmarchicus distribution, abundance,
and diapause arousal timing in waters adjacent to GB/GoM.
The C. finmarchicus
biological simulations (experiments 1-3) are complementary to already funded Calanus modeling projects (Franks et al. project) that are more specific
to the dynamics of Calanus during the
active season in the GoM/GB and Scotian Shelf regions. Our project focuses on the transports
and physiological awakening of Calanus
during their deep-dwelling diapause phases and on the implications of NAO
variability and cold Labrador Sea influence on
the population dynamic timing and ability of C. finmarchicus to exploit the strongly seasonal production cycles
typical of temperate continental shelf habitats.
Significance and Intellectual
Merit. This project will develop a quantitative understanding
of how North Atlantic basin-scale forcing
(NAO), acting through variable water mass formation and water transports,
impacts the physics, nutrient chemistry and ecology of the Northwest
Atlantic. Our investigation focuses on the ecological impacts of
variable inflow of cold, fresh, nutrient-poor water from the Labrador
Sea to the NW Atlantic, because historical records suggest that
inflow events of both short duration (like 1998) and longer duration (1960's)
occur and ecosystem productivity of the region, as observed in Calanus finmarchicus abundance, has
responded to these changes.
To address variability over these multiple time scales,
we propose to do the following during the two-year duration of the project: (1)
Set up and run an individual based model (IBM) for the Northwest Atlantic,
using the high-NAO (1980-1993) and low-NAO (1962-1971) forced physical fields
from an ongoing eddy-resolving North Atlantic simulation to understand
multi-decadal variability of Calanus
finmarchicus seeding and production in this region; (2) Perform a set of
eddy-resolving basin-scale model simulations during 1988-1999 starting from
already existing high-NAO simulations (from an ongoing NASA project) and run
the IBM to study the interannual variability of C. finmarchicus seeding and production in this region; (3) Analyze
long-term in-situ physical and
biological datasets and satellite-derived sea surface temperature (SST) along with
in-situ physical, biological, and
chemical data collected during the GLOBEC core-measurement period (1995-1999),
and validate the two basin-scale physical and biological fields to develop a
broader understanding of C. finmarchicus
seeding and production; and (4) Generate four-dimensional high-resolution
(5-km) physical fields using basin-scale fields and available data during
1993-1999, and run a series of IBM simulations at higher resolution to address
questions relating ecosystem variability on the Scotian Shelf, on Slope Sea and
within the Gulf of Maine and on Georges Bank to the large-scale fluctuations of
the NAO.
Broader Impacts. We
anticipate that our work with satellite-derived SST data under this proposal
will provide a synthetic focus to assist with other regional observational and
modeling work being proposed by other groups. Our
study on the impact of large-scale climatic and basin-scale forcing on the
regional ecosystem of the GoM/GB region will enhance the understanding of the
larger community. We will train two graduate students during the two years of
this project. Results will be disseminated by peer-reviewed scientific
publications and presentations at national conferences. We will make the model
output available via a website linked to the GLOBEC website. We will give
public lectures at Dartmouth
High School on the
importance of NAO and its impact on the regional ecosystem as part of our
ongoing K-12 outreach program.