Progress Report: April 1999
Project Title: Linked Biophysical Modeling in the California Current
System: The Influence of Circulation and Behavior on Prominent
Mesozooplankton Species
Investigators: Thomas (Zack) Powell (PI), Hal Batchelder (Research Scientist),
Chris Edwards (Post-Doc.), (All at Univ. Calif. Berkeley), and Dale Haidvogel (PI) and
Mohamed Iskandarani (Asst. Res. Prof.) (Both at Rutgers University) (
This research developed detailed understanding of coupled
physical/ecosystem models in relatively simple contexts in anticipation of
more complete and complicated three-dimensional simulations of the
California Current System (CCS). Our approach emphasizes a hierarchy of
model capabilities, progressing through a series of numerical experiments
of increasing complexity in one and two physical dimensions. The flowchart
(Figure 1) depicts our strategy in linking physical, ecosystem and
individual based models.
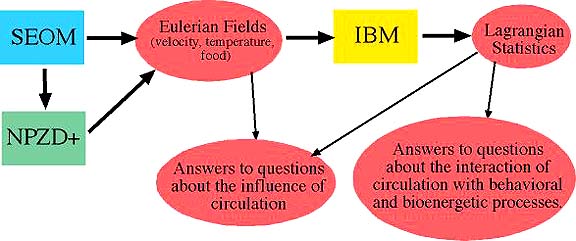
Figure 1. Flow-chart showing model interactions and how they contribute to
our fundamental research questions.
Two-Dimensional Upwelling Ecosystem Study
We constructed a two-dimensional (x-z, vertical slice), finite-difference
hydrodynamic model, subject to vertical mixing parameterized using the
Large et al. (1994) algorithm. Physical fields were used to drive the
Franks et al. (1986) NPZ ecosystem model. Conventional application of this
ecosystem model (Franks and Walstad 1996; Wroblewski et al. 1988) assumes
that macrozooplankton are the dominant herbivores. However, recent
evidence (Strom and Morello 1988; Strom et al. 1993; Verity 1985)
illustrates the substantial contribution of microzooplankton to the
nitrogen cycle, and our approach examines how a quintessential ecosystem
response changes when this arguably more realistic parameter range is
considered. We modeled the coast off Newport, OR, during idealized
upwelling-favorable wind conditions. Coastal upwelling injects nutrient
rich water into the euphotic zone, and induces the development of
phytoplankton blooms immediately offshore in both macro and
microzooplankton scenarios. The vertical extent of primary production is
controlled by the surface mixed layer physics. However, the horizontal
scale of the bloom is determined by the biological dynamics specific to the
parameters applied. Microzooplankton have rapid growth and respond quickly
to phytoplankton blooms, shortening their duration, which in an offshore
Ekman drift yields a limited zonal bloom. In addition to the quantitative
changes to the duration of the phytoplankton maxima, qualititative changes
in the model response are evident in the biological fields downstream.
Specifically, the microzooplankton parameterization drives a roughly 20 day
oscillatory behavior manifested as a narrow zooplankton maximum just
downstream of the phytoplankton peak, with low biomass further offshore.
Over long time-scales, this behavior leads to phytoplankton patchiness,
both spatially, depending on the duration of the wind-stress forcing, and
temporally, in the case of wind-relaxation. In contrast, the overdamped
pendulum-like response of the macrozooplankton parameter model leads to a
slow return to a sizeable steady zooplankton level offshore, and no
phytoplankton patchiness. Denman and Abbott (1988) argue that biological
productivity is predominantly determined by physical processes, but our
model exemplifies a case in which biological processes play a fundamental
role setting the spatial structure of the fields (Abbott and Letelier
1998). Results from this research have been presented at two conferences
and are in preparation for a peer-reviewed journal (Edwards et al. 1999).
Effect of Realistic Ocean Mixing on NPZ Oscillations
The purpose of this work was to characterize the stability of the Franks et
al. (1986) NPZ model in the presence of vertical mixing. Ecosystem models
of the NPZ type are coupled nonlinear systems of equations that exhibit a
well-known range of dynamical behavior from stable equilibria to limit
cycles to chaos. The dynamical regime of a particular model is of course
determined by the parameter set applied. What is not generally discussed is
that even with a particular parameter set, some components of the model
(e.g., uptake rate) are spatially dependent. This variation can lead to
different dynamical regimes within the water column (Figure 1). We have
performed a linear stability analysis of the Franks model and shown that
both stable fixed points and limit-cycle dynamics are common features of
even a single vertical profile. However, the addition of diffusion
modifies this stability, by coupling the otherwise independent oscillators
and stable points. For some parameter sets (e.g., the macrozooplankton
parameters discussed above), this coupling stabilizes the vertical profile,
whereas for others the dynamics remain unstable (e.g., the microzooplankton
case). For still other (quite plausible) parameter sets, the dynamical
regime of the coupled system can vary non-monotonically from stable to
unstable states depending on the magnitude of the diffusion. Indeed, for
very weak levels of diffusion, the model exhibits chaotic dynamics for both
parameter sets explored, though this state does not appear for more
realistic, natural levels. Understanding the dynamical regime of the model
in the presence of mixing has important implications for the appropriate
initialization of biological models, as well as toward the interpretation
of numerical integrations in more complicated advective scenarios. This
effort has led to the preparation of one paper (Edwards and Powell 1999)
for a peer-reviewed journal and presentations at two academic conferences.
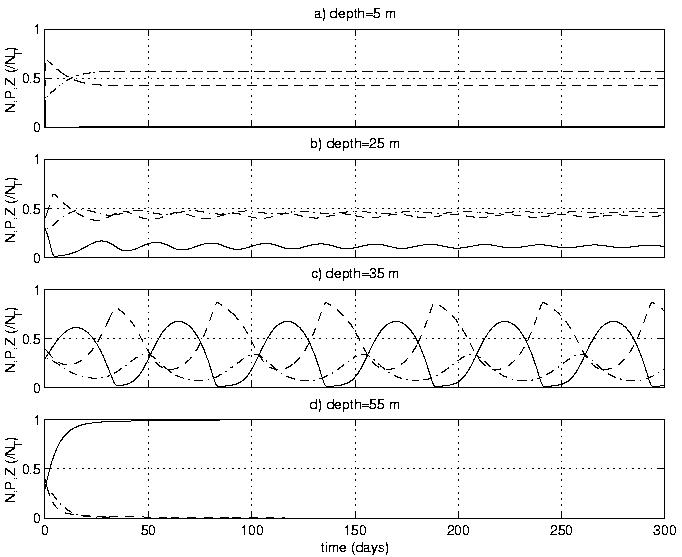
Figure 2. Time series at 4 depths using the Franks et al. (1986) model and
macrozooplankton parameters. Model dynamical state varies with depth.
Phytoplankton (dash-dot); Zooplankton (dashed); Nutrient (solid).
Individual-Based Modeling of Metridia pacifica
We coupled 2D Eulerian biophysical simulations of hydrodynamics and lower
trophic levels (NPZ models) with Lagrangian particle tracking IBMs of
larger zooplankton in the California Current ecosystem. Our primary goal
was to evaluate how the interaction of extrinsic (environmental) and
intrinsic (specific to the individual organism) factors control
distribution and demography. We focused on the interactions of food
concentration, advection, diffusion and light (extrinsic factors) with
organism size, nutritional state, and behavior (intrinsic factors) in the
copepod, Metridia pacifica. Extensive experimental data and field
observations exist (Batchelder 1986a) for this species, and it has been
modeled previously in 1D (Batchelder and Miller 1989; Batchelder and
Williams 1995). We extended earlier population dynamics models in two
respects: 1) by forcing the IBM using physics and food resources obtained
from an idealized 2D Eulerian simulation; and, 2) by exploring more
realistic, mechanistic models for diel vertical migration (DVM). We
developed and tested a particle tracking model (PTM) to couple the Eulerian
fields from the slice model to the Lagrangian IBM. The PTM moves animals
by advection, diffusion, and vertical migration. The latter is affected by
ambient light intensity and food concentration, proximity to the bottom and
surface, and individual size and nutritional state.
We have identified four metrics for evaluating the response of organisms to
their environment: 1) growth/development; 2) reproduction; 3) survival;
and, 4) distribution, particularly nearshore retention. For an individual
to be successful in an eastern boundary current upwelling system it must
grow, reproduce and survive. Moreover, since ocean productivity is
greatest nearshore, zooplankton production is enhanced by nearshore
retention. We examined growth and nearshore retention only. Suitable
habitat space is the region of the domain that favors nearshore retention
and positive individual growth. Model results, using an idealized
upwelling circulation, indicate that DVM interacts with the 2D flow fields
to retain individuals nearshore when the amplitude of the vertical
excursion places animals in near-bottom onshore flow during the day (Figure
3). This occurs more often and from a larger "volume" of the upwelling
system for larger organisms than for smaller individuals, and is most
effective deep and inshore (<40 km from shore). The spatial extent and
magnitude of the phytoplankton bloom (food resources) strongly controls the
volume of suitable habitat space for the copepods. Spatially-extensive and
high concentration blooms (macrozooplankton scenario) provided a much
larger suitable habitat than did smaller blooms (microzooplankton
scenario). Rapid growth from small, nonmigratory life stages to larger,
migrating stages provides greater opportunity for nearshore retention. This
indicates that resource fields are important in determining not only growth
rates, but also nearshore retention, and thus, argues for detailed
descriptions of how resources vary in space and time. This research has
been presented at one conference and one invited talk. Future work will
extend the model to other targeted species (Calanus pacificus and Euphausia
pacifica).
More detailed information about the IBM simulations and movies of results are available here.
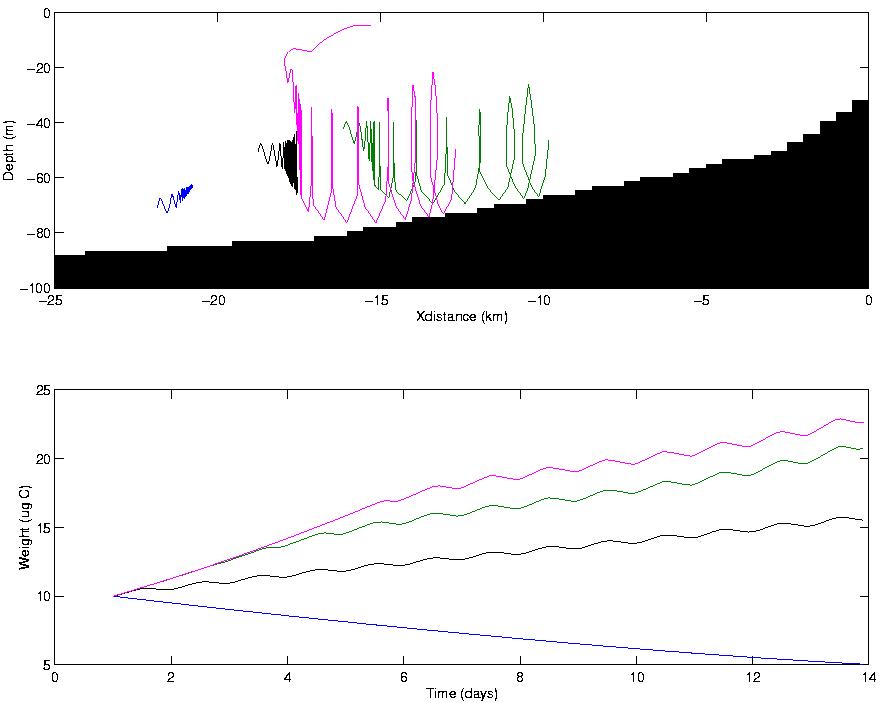
Figure 3. The upper panel shows 13-day trajectories of four individual copepods in
the nearshore region within an upwelling circulation. Three individuals
nearest to shore experienced positive growth (lower panel). The individual
located deep and furthest from shore experienced negative growth leading to
mortality. Weight fluctuations result from size-dependent diel vertical migration between low (deep)
and high food regions (shallow).
Large-Scale Physical Modeling
Two models initially developed at Rutgers University are in wide use within
the U.S. GLOBEC program. They are the Regional Ocean Modeling System
(ROMS) and the Spectral Element Ocean Model (SEOM). ROMS is the latest
version of the S-Coordinate Rutgers University Model (SCRUM), recently
reconfigured in collaboration with colleagues at UCLA for improved
algorithmic performance and accuracy. System attributes include extensive
restructuring for sustained performance on SMP-class parallel-computing
platforms; high-order, weakly dissipative algorithms for tracer advection;
a unified treatment of surface and bottom boundary layers, based on the
Large et al. (1994) and Styles and Glenn (1999) algorithms; an integrated
set of procedures for data assimilation (nudging, optimal interpolation,
and the reduced-state Kalman filter); and advanced treatments of open
boundary conditions. Coupled physical/biological models based upon
SCRUM/ROMS are now in place in the Coastal Gulf of Alaska in support of
GLOBEC NEP (see, e.g., Hermann and Stabeno 1996).
The primary engine for simulating the three-dimensional circulation of the
Northern California/Oregon coastal region will be the spectral element
ocean model (SEOM) developed by Iskandarani and Haidvogel (see below).
Prior support from U.S. GLOBEC has enabled the continued development of
SEOM, and its evaluation in both idealized and realistic, basin-scale
settings. Recent technical improvements to the three-dimensional
circulation model fall predominantly in the areas of sub-gridscale
parameterization and multi-scale nesting/coupling. In the former area, the
introduction of spatially adaptive horizontal diffusion operators and
filters now allows scale- and process-selective viscous closure. In the
vertical, the KPP (Large et al. 1994) vertical mixing algorithm has been
implemented on the native (finite element) grid of SEOM, and shown to
reproduce known mixed-layer responses to prescribed applied surface fluxes.
Nearshore resolution for the Northeast Pacific regional SEOM model will be
less than 5 km (Figure 4).
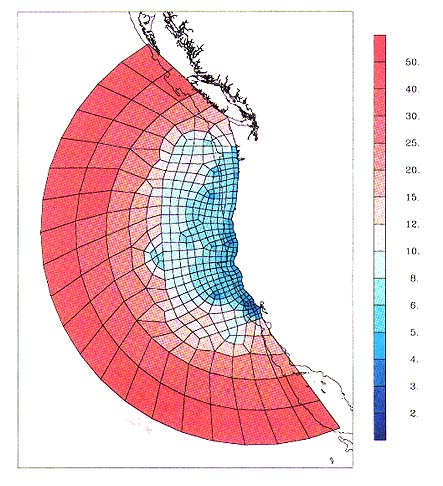
Figure 4. Elemental boundaries of Northeast Pacific regional SEOM grid. Colorbar indicates horizontal resolution (km).
Importantly for our GLOBEC applications, SEOM now offers a unified
treatment of inter-regional (multi-scale) coupling, including in a single
framework the capabilities for continuously variable grids, model/model
nesting, and regional open boundary conditions. An important recent
development is the implementation of an inter-grid nesting capability based
on the mortar element method, which allows two-way exchange of information
between grids of differing elemental partition and/or spectral truncation.
As a proof-of-concept of this mortar element capability, we are currently
testing a two-way coupled version of a SEOM-based North Atlantic Basin
model with the GLOBEC Georges Bank model developed at Dartmouth College by
Prof. Dan Lynch.
Finally, intercomparative testing of numerical models -- including MOM and
MICOM, as well as SEOM -- has shown the considerable advantages of the
higher-order SEOM code in the limit of idealized, process-oriented test
problems, including the large-scale wind-drive ocean circulation, flow
around coastal canyons, and gravitational adjustment and downslope flows.
Two peer-reviewed publications (Levin et al. 1998; Curchitser et al. 1998),
and a forthcoming monograph (Haidvogel and Beckman 1999), describe our
progress in these areas.
Publications
Curchitser, E. N., D. B. Haidvogel, and M. Iskandarani. On the transient adjustment of a mid-latitude basin
with real geometry: the constant depth limit. Dyn. Atm. Ocean. (Submitted).
Edwards, C. A., H. P. Batchelder, and T. M. Powell. On microzooplankton and macrozooplankton dynamics within
an upwelling ocean ecosystem. (To be submitted to Marine Research)
Edwards, C. A., and T. M. Powell. The stability of a NPZ model subject to realistic ocean mixing. (To be
submitted to Marine Research)
Haidvogel, D. B., and A. Beckmann. 1999. Numerical Ocean Circulation Modeling. Imperial College Press, London.
Levin, J. G., M. Iskandarani, and D. B. Haidvogel. A non-conforming spectral element ocean model. Int. J. Num. Meth.
Fluids. (Submitted)
Stacey, M. T., H. P. Batchelder, T. M. Powell, and S. Twombly. Estimation of biological vital rates using an augmented Kalman
filter. (Submitted to Limnol. Oceanogr.)